What are the best composite metal coatings for medical devices and implants?
10 February 2021Medical devices and implant numbers have grown to the extent that there are over 1,700 types of device and 50,000 approved medical devices in the US alone, according to the US’s National Center for Biotechnology Information. They vary in complexity from tongue depressors to complex imaging devices, such as magnetic resonance imaging (MRI) machines.
Within this range of complexity, there is often much more to what appears to be a simple device than meets the eye. A seemingly simple titanium prosthetic joint, prized because of the light metal’s unique characteristics, will need microscopically accurate machining and complex composite surface coatings to ensure it improves outcomes for its user.
Similarly, complex automated test equipment may feature multiple light and valve metal alloy components to benefit from properties such as high strength to weight ratios, but also require wear and corrosion resistance, amongst other characteristics.
There are a range of composite surface coatings that enhance the natural properties of light and valve metals. These enable medical device manufacturer (MDM) designers not only to exploit the existing benefits of using light and valve metals and their alloys, but to augment them, too.
Materials’ surface properties are required for medical applications
When designing new or updating existing medical devices and implants, designers are increasingly having to meet higher standards, both regulatory and in terms of advances in medical procedures.
Surface coatings act as enablers to the light and valve metals, such as aluminium, titanium, niobium, zirconium, tantalum and hafnium. They can enhance these metals’ properties so they can be used in applications where, without the coating, they would not be suitable. The properties designers need to look for in a light metal and its surface coating include:
Wear resistance: titanium is the favoured solution for artificial limbs and joint replacements but suffers from wear. Wear analysis is a vital function of implant manufacturers, so they can understand exact mechanics causing wear. This is not ideal for joint replacements, where two metals slide against each other. Engineers require materials that resist abrasive and adhesive wear, all so they can provide the most long-lasting treatment for patients.
Hardness: as above, artificial joint replacements are in constant contact so must have sufficient hardness to resist wear and scratching, but also deformation, indenting and penetration.
Corrosion resistance: the human body is a hostile environment for many materials, as are the many types of treatments used to sterilise medical devices. Unprotected materials will rapidly corrode in steam autoclaves or when harsh disinfectants are used in healthcare environments.
Thermal / heat resistance: steam autoclaves (for sterilisation) reach temperatures of up to 135 degrees centigrade to deactivate harmful microorganisms. This does not affect most metals in the short term but can cause heat fractures over the long term. Many plastics will melt at those temperatures.
Hydrophilic / hydrophobic: simply put, a hydrophobic material rejects liquids and a hydrophilic material attracts liquids. Hydrophobic properties promote osseointegration of implants, as well as contributing to corrosion resistance in some applications.
Antimicrobial: many materials have antimicrobial properties, such as silver and copper that kill microorganisms, polymers and paints that do not allow bacteria to adhere to surfaces and form colonies, and photocatalytic surfaces that kill microorganisms when activated by light, usually in the ultraviolet spectrum.
Biocompatibility: this is relevant to implants and devices that are used within the body. The surrounding tissues must accept an implant without it, for example, causing an immune response, allergic or inflammatory response or cancer. The surface of the implant must not lose material or create chemicals through, for example wear or corrosion, that will damage the body.
Composite surface coating applications
Asking for a material and surface coating that has all the above properties is a tall order. In practice, a medical device or implant would not require all the above properties. However, by using composite or duplex coatings, it is possible to combine several surface coatings to deliver a range of properties required for medical applications
Chemical vapour deposition (CVD) is a surface coating technology that deposits extremely thin films of the coating onto the substrate, as the reagents are flowed over a high temperature substrate. The substrate is heated to 600-1100 degrees centigrade, causing the reagents in the gas to react to form a thin film coating, which sometimes includes the substrate, depending on the reagent. The advantages of the approach include specifying the reagents to deliver coatings with different properties. Furthermore, because the reagents are a gas flowed around the substrate, it gives a consistent coating, unlike line-of-sight methods. Finally, when used to deposit polymer thin films via CVD polymerisation, multi-layered CVD is used for pharmaceutical and antimicrobial antifouling applications.
Thermal spray coatings can add a coat of anything that can be melted, via flame, plasma or electric arc spraying. Composite coatings with multiple properties can be created by combining several coating substances. The coating is formed as a gun sprays the substrate with the coating material as droplets, which mechanically bond to the substrate. Although used for biocompatible coatings on medical implants, the technique is line-of-site so the coating may not be consistent. Furthermore, there is no bond with the substrate surface, which must be specially cleaned and roughened before coating.
Magnetron sputtering deposition is a form of physical vapour deposition (PVD) that uses strong electromagnets to control how sputtered atoms from the source material are deposited onto the target substrate. The advantage of this surface coating technology is the precision control that materials engineers have over the structure and thickness of the coating. The composition of the source gas can be varied to tailor the composition of the surface coating, depending on the needs of the end application. Anti-microbial textiles feature composite layers of copper, zinc and titanium applied by magnetron sputtering deposition.
Plasma electrolytic oxidation (PEO) uses an electrolyte bath and plasma to form a ceramic-like multi-later coating on light and valve metals and their alloys. The multi-layered coating’s top layer is also highly porous. In addition to forming the base layer from the substrate so there are no bonding issues, uniquely, PEO incorporates the contents of the electrolytic bath in the top porous layer of surface coating. As a result, it is the perfect surface coating technology that can, for example, introduce a photocatalyst such as TiO2 into the porous layer. The top layer can be further customised with other reagents to introduce greater corrosion and wear resistance, hydrophobic/hydrophilic properties and thermal barrier coatings. Because the layers are formed from the substrate, and incorporate the electrolyte bath components into its microstructure, there are no bonding issues, and the surface does not flake or leak ions. As a result, biocompatibility is high.
To learn more about PEO, download our white paper ‘What is Plasma Electrolytic Oxidation?’ or get in touch with one of our materials scientists today.
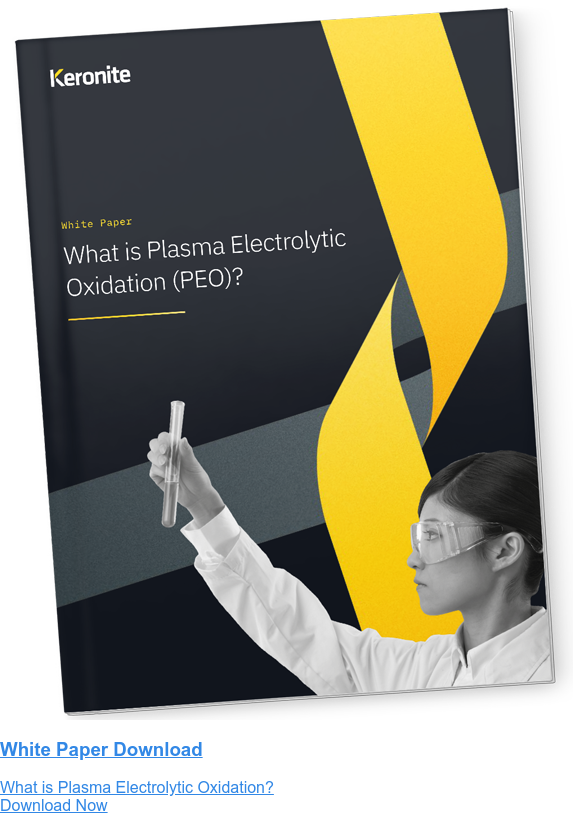
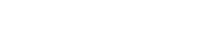